The past couple of years I and many others in the energy world have become fascinated with thermal energy networks, or, as us nerds call them, "TENs."
In my last series on energy (part one, part two, and part three), I didn't really touch on them because I was focused on more general questions of electrical energy. TENs, importantly, bring our attention down to the level of how to use that electricity for a very particular use: heating and cooling.
Heating and cooling, globally, are a massive and growing part of our global energy use. In 2018, the International Energy Agency estimated that 20% of all end-use energy goes towards heating and cooling, and that the 2.8 billion people in the world's hottest areas will be hungry for more than double the cooling the world has now as they get wealthier – and things get warmer.
In wealthy countries where heating and cooling are already widespread, the evolution will look different. As detailed by friends at the Building Decarbonization Coalition, there's a huge movement to help existing gas networks for heat pilot ways to transform into renewably powered TENs.
Because of their cost-effective, resilient, and energy efficient features, I think 2025 will be a breakout year for talking about – and hopefully building – TENs systems around the world.
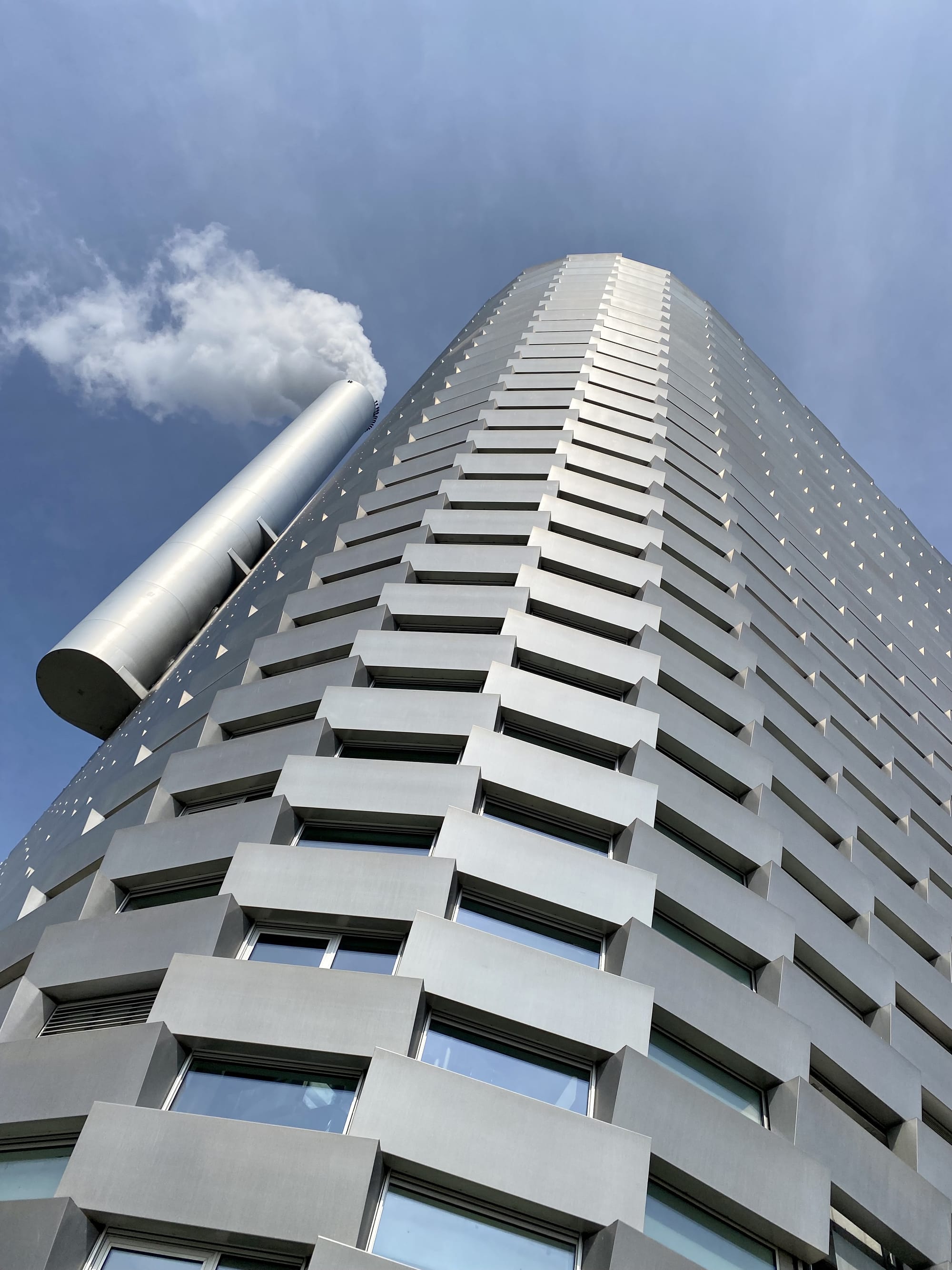
The Maze of Energy Systems Terminology
Thermal energy networks are one term of art that is coming to significance amidst a field of other, related and generally overlapping concepts. Before I even get into the specifics of these district systems, let's start from the very top:
Every aspect of our lives is connected into an energy system, a collection of technologies, such as transmission lines and nuclear power plants, laws, regulations, and standards (e.g., those created by the International Electrotechnical Commission), and local physical contexts (e.g., BC is a hydro-electricity biased jurisdiction).
Energy systems, as I explored with reference to RMI's work in the first part of my energy series, deliver energy services that respond to two fundamental human needs:
- The need for work-energy to do stuff in the world.
- The need for heat services (i.e., heating and cooling).
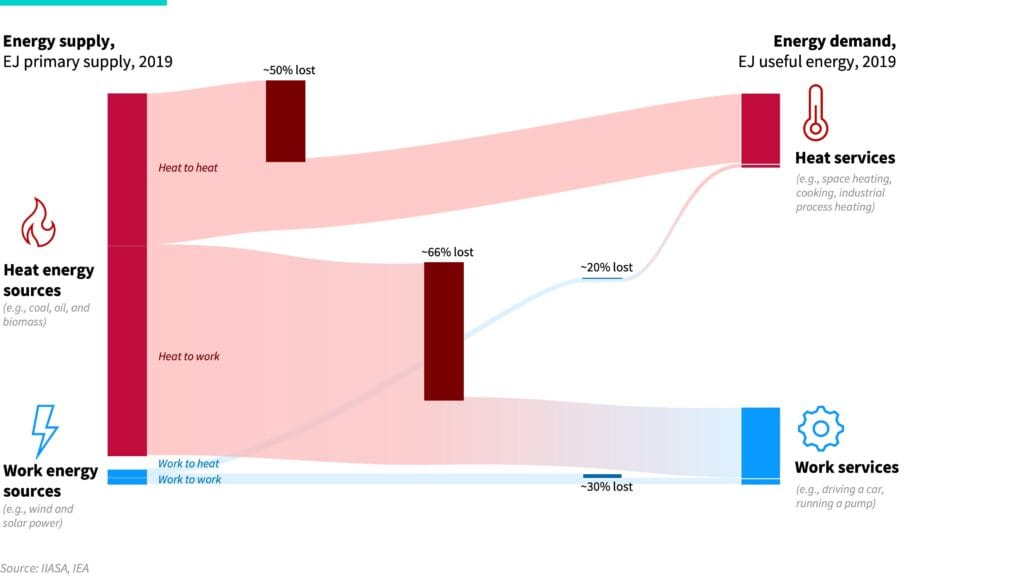
You can supply this energy in centralized (e.g., a nuclear power plant sends electricity to a whole city from one place), or decentralized (e.g., every house has rooftop solar and gets power from somewhere else) ways.
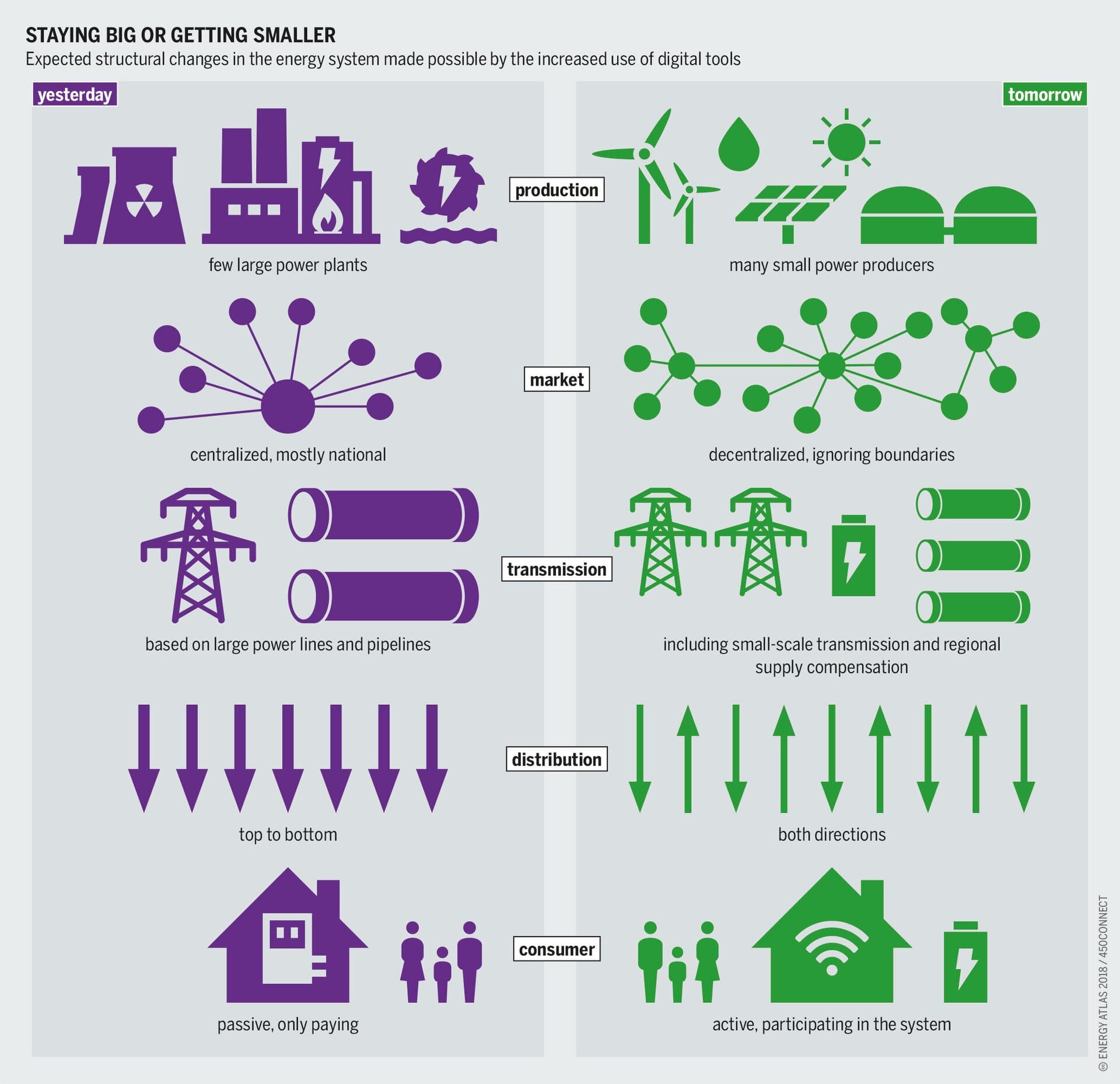
As the demands on our electricity system become (a) larger and (b) more complex, moving towards more distributed approaches is becoming a more cost-effective, resilient, and fair way of managing our needs. We increasingly call this collection of specific technologies and configurations of those technologies distributed energy resources, or, again, for the nerds, DER.
DER, like TENs, is having something of a moment right now. David Roberts did a brilliant feature on them early in 2024 and since then it feels like the pop up in every conversation I have on energy.
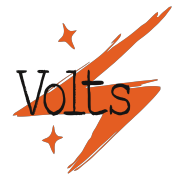
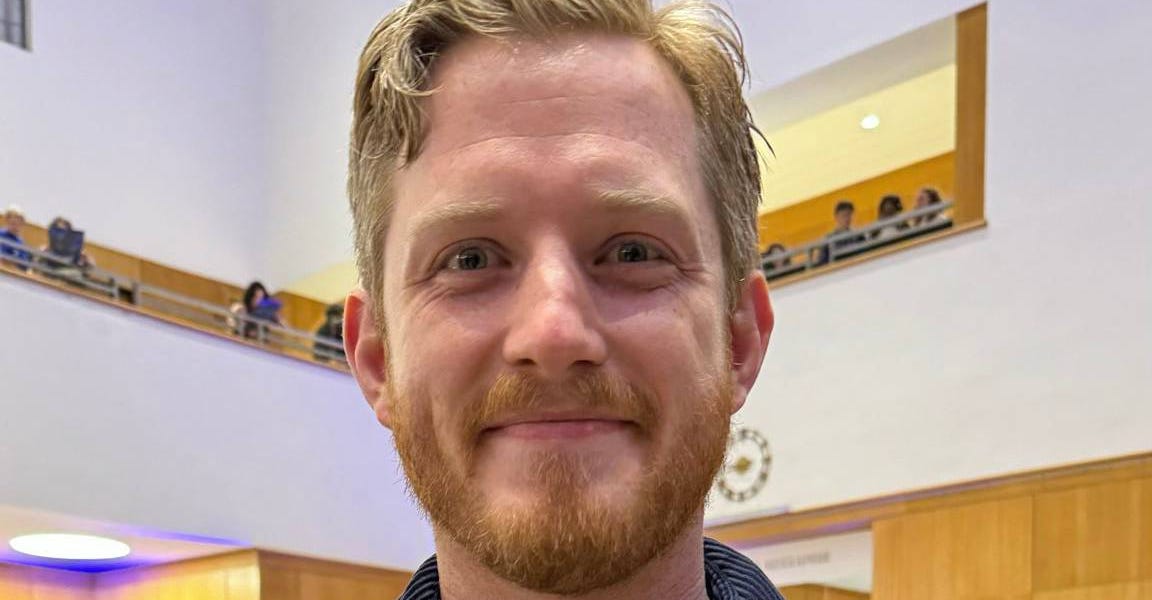
Distributed energy resources are the widest possible way of thinking about systems which, as the name suggests, are not top-down, one-way approaches to delivering the work or thermal comfort that people need. The DER approach we're interested in this article is, obviously, thermal energy networks.
Here's where the terminology gets sticky:
TENs are often used synonymously with district energy systems (DES). District energy systems are generally considered the higher-order category because they can include combined heat and power (CHP) systems, where a facility produces, as the name says, both heat and power – because heat doesn't travel well, these systems are generally localized, hence being considered part of the 'district' systems.
Thermal energy networks are a subset of these district systems that focus on providing heating or cooling (and increasingly both) to a specific local area. As the Building Decarbonization Coalition defines them:
Thermal Energy Networks (TENs) provide efficient and affordable clean energy heating and cooling to entire neighborhoods through a shared network of water pipes that transfer heat in and out of buildings. These neighborhood-scale systems allow buildings to exchange heat with a number of energy sources, such as lakes and rivers, energy intensive buildings, wastewater systems, or the stable temperature of the earth, and can be designed with backup systems to remain reliable even amid a power outage. TENs can also use shallow boreholes to capture and store excess heat underground for use days or months later.
With me so far? Hopefully, but here's where it gets a little more complicated.
To explain the full range of types of TENs, we need to explore a little history.
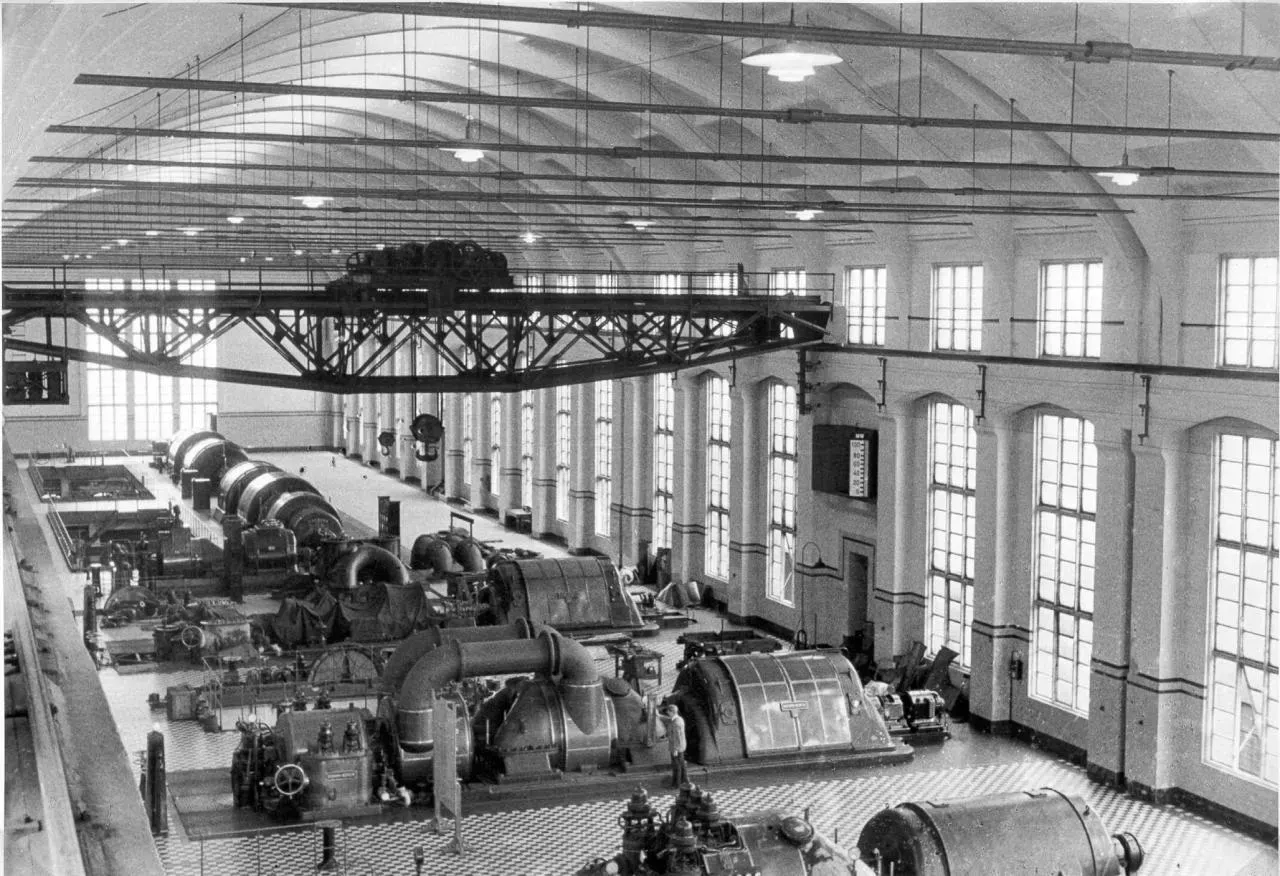
The Actually Cool History of District Energy
Remember how I said there are centralized and decentralized energy systems? That's also true within TENs, just at a much smaller scale.
The earliest systems were completely centralized, with one, moving hot steam or water from one place, to a few nearby buildings. In the most modern systems, you can find things like an "ambient loop" structures which radically decentralize where heat comes into the system, is stored, and balanced between different users.
While there are a range of examples going back to Ancient Rome, the first modern district heating system, which was very much centralized, was developed by Birdsill Holly in New York, in 1877. As the American Society of Mechanical Engineers writes:
As a means of avoiding the basic inefficiency of heating buildings with individual small boilers, Birdsill Holly invented the "district" steam-heating system. Its basis was a large central boiler plant that furnished steam under moderate pressure to a group of buildings in a surrounding district through a loop of supply and return mains, heavily insulated to reduce heat loss. Each customer was charged for the amount of steam consumed, determined by metering the water of condensation.
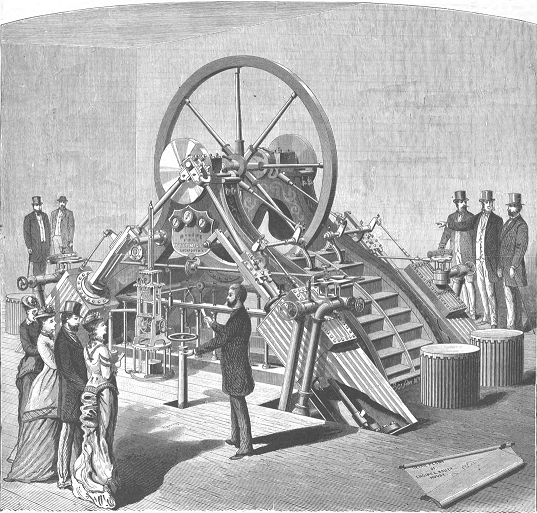
There's a whole industry and technical debate about a series of "generations" of different systems that came after the 1870s. Some people really hate it because it gets into some pretty specific hair-splitting, but it's helpful to know it in broad strokes.
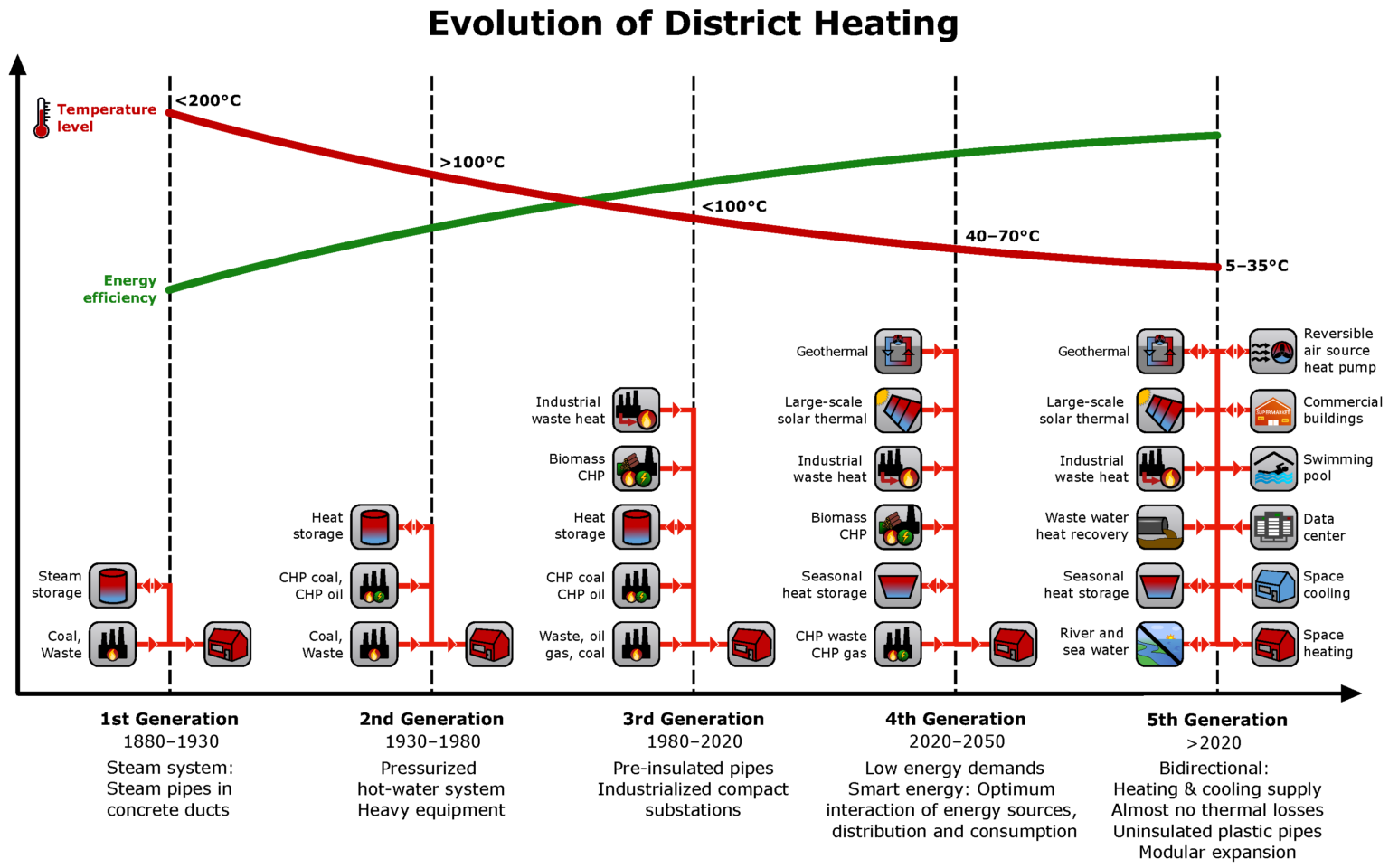
- 1870s-1930s – First generation systems were systems that distributed steam from a central plant to one of a few buildings within a district, the water to create the steam having been heated by a coal-fired boiler.
- 1930s-1980s – Second generation systems involved pressurized water moving through sealed pipes, involving more modern coal-boiler systems, coal-, and later oil-powered combined-heat and power systems.
- 1980s-2020s – Third generation systems, sometimes called "Scandinavian district heating" because of their dominance in that region, are effectively a more modernized version of the second, with more pre-fabricated parts, insulated, specialized pipes, with the ability to heat water to a lower temperature (and therefore be more energy efficient) because of the lower risk of losing heat along the way.
- 2020s+ – Fourth and fifth generation systems are extremely heterogenous, with mixtures of all of the old prior systems, with the addition of (for fourth) geothermal and solar-thermal technologies, and in the case of fifth generation, drawing on waste heat from large buildings and data centres, with added industrial, reversible heat pumps.
I like to simplify all of this to: as district heating and cooling network advance,
- The number of technologies involved in a network increases, from what supplies the heat (e.g., from coal to heat pumps), to how its distributed.
- The necessary temperatures for the network decrease, first for steam, and then water, from <200C in the 1880s, to theoretically as low as 5C.
- The diversity of connections within a system increases, first from industrial systems and later to complex combinations of natural sources (e.g, geothermal) and smaller anthropogenic (e.g., swimming pool waste-heat) of heat.
- They integrate more and more diverse forms of thermal storage, allowing heat to be saved (increasingly across seasons), preventing waste and therefore reducing energy inputs.
- The networks become multi-directional, where heating and cooling are traded between users within a single district, reducing overall energy inputs.
- The networks involve more participation by end-users (e.g., homes) in balancing heating and cooling, usually with different sizes of heat pumps.
It's important to say here that this evolution is not a straight line towards progressively "better" systems. The whole point of fourth and fifth generation systems is that they represent an extremely high level of sophistication and often a mix of technologies that can be tightly adapted to a given context.
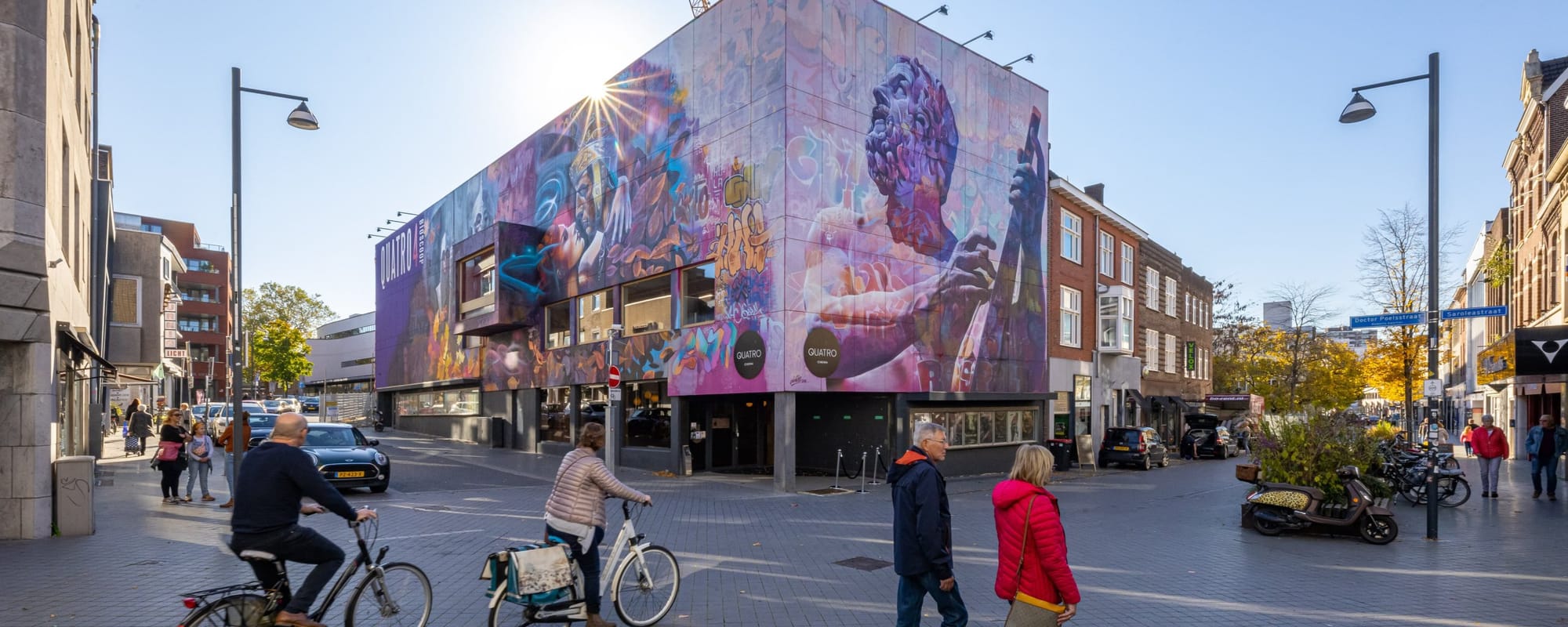
Where are we going from here?
In their great conceptual article differentiating fourth and fifth generation systems, Henrik Lund and his colleagues identify that the main ideas defining fifth generations systems are that they:
- Take advantage of the synergy of combined heating and cooling in areas of mixed purpose buildings,
- Minimize the barrier of utilizing local waste heat sources and minimize upfront investment cost for the utility company, though the required initial investment at the end-users will be higher, and
- Enable less restrictive organic growth of the system, as central heat supply is not as critical, since new additional end-users will both add and use heat from the network.
This means you can get complex, multidirectional flows of hot and cold water being moved around, heated and cooled, fed into heat pumps, and stored.
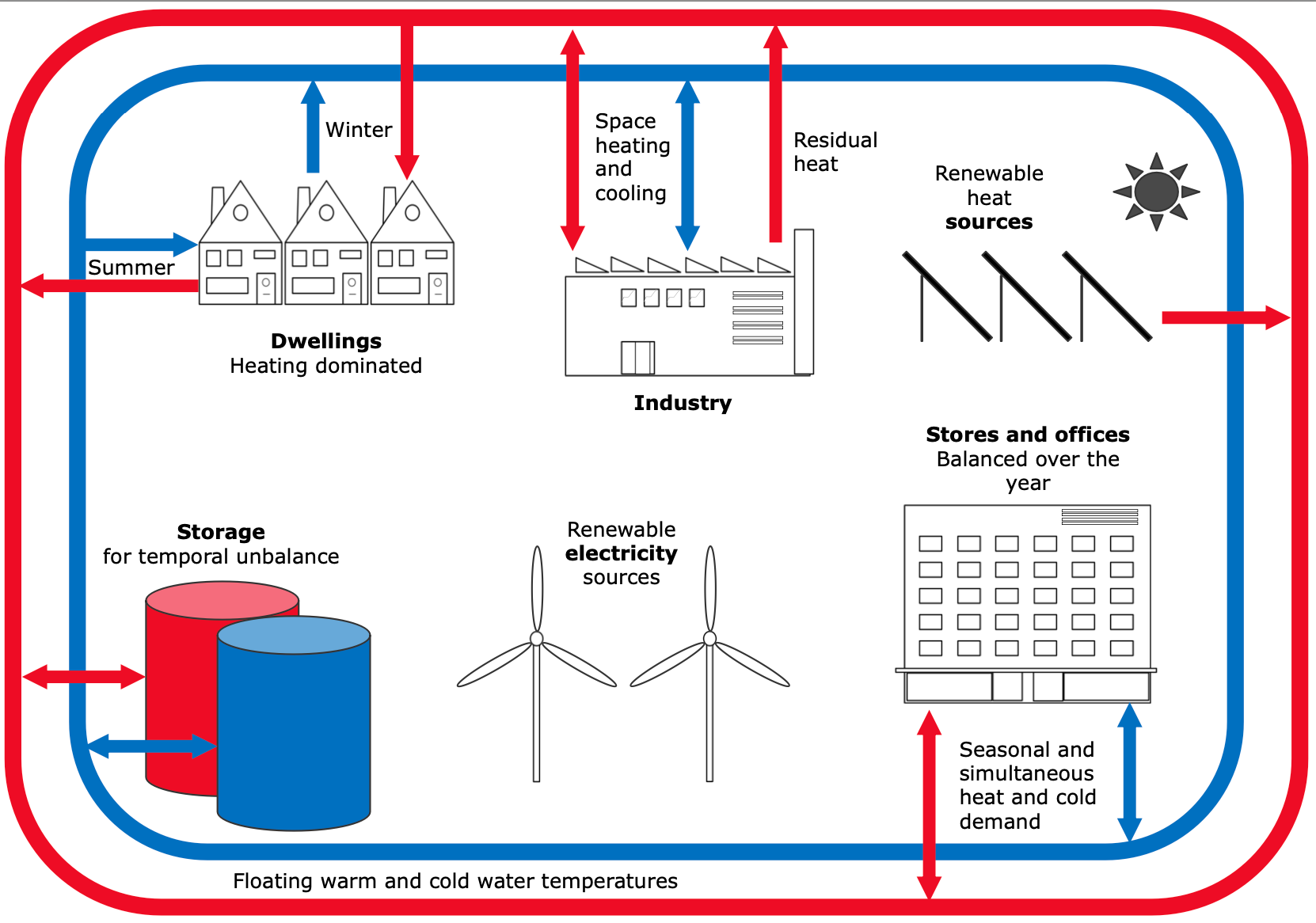
This is exemplified in systems like the Mijnwater project in Heerlen, in the Netherlands, or the many, many projects underway in Germany.
These are highly complex systems and, as they're so novel and innovative, it seems likely that more than a few will face challenges, and some may even fail. But the mixture of these newer types of systems, and the tried and true older third and fourth generation systems will continue to deliver heating and cooling to more and more people around the world.
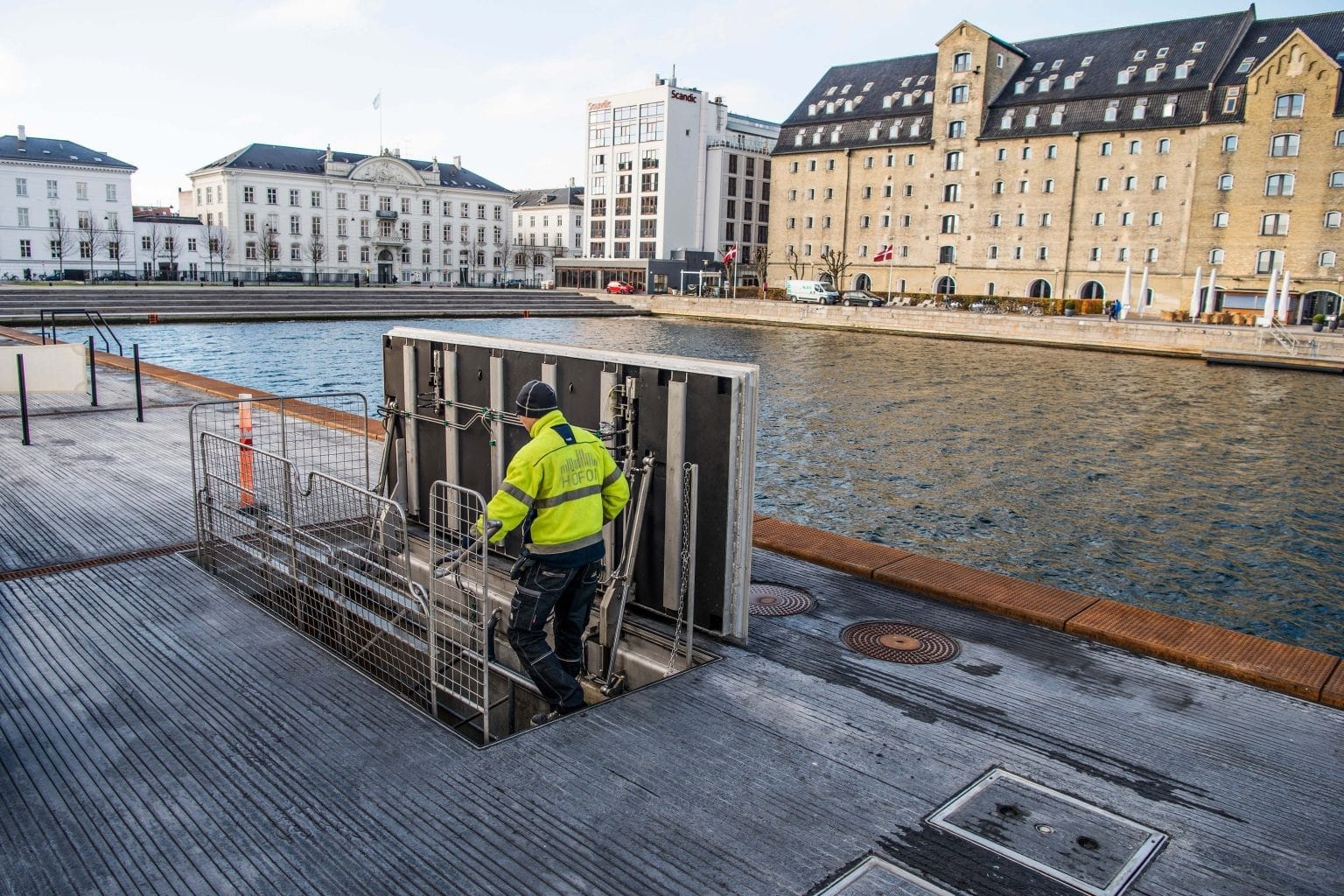
Thinking bigger about our future energy system – by thinking smaller
As I said at the start, heating and cooling where we live, work, and play is going to be one of the single most important energy issues of the next century. This is especially true in a changing climate. The IEA data I mentioned at the start makes that abundantly clear.
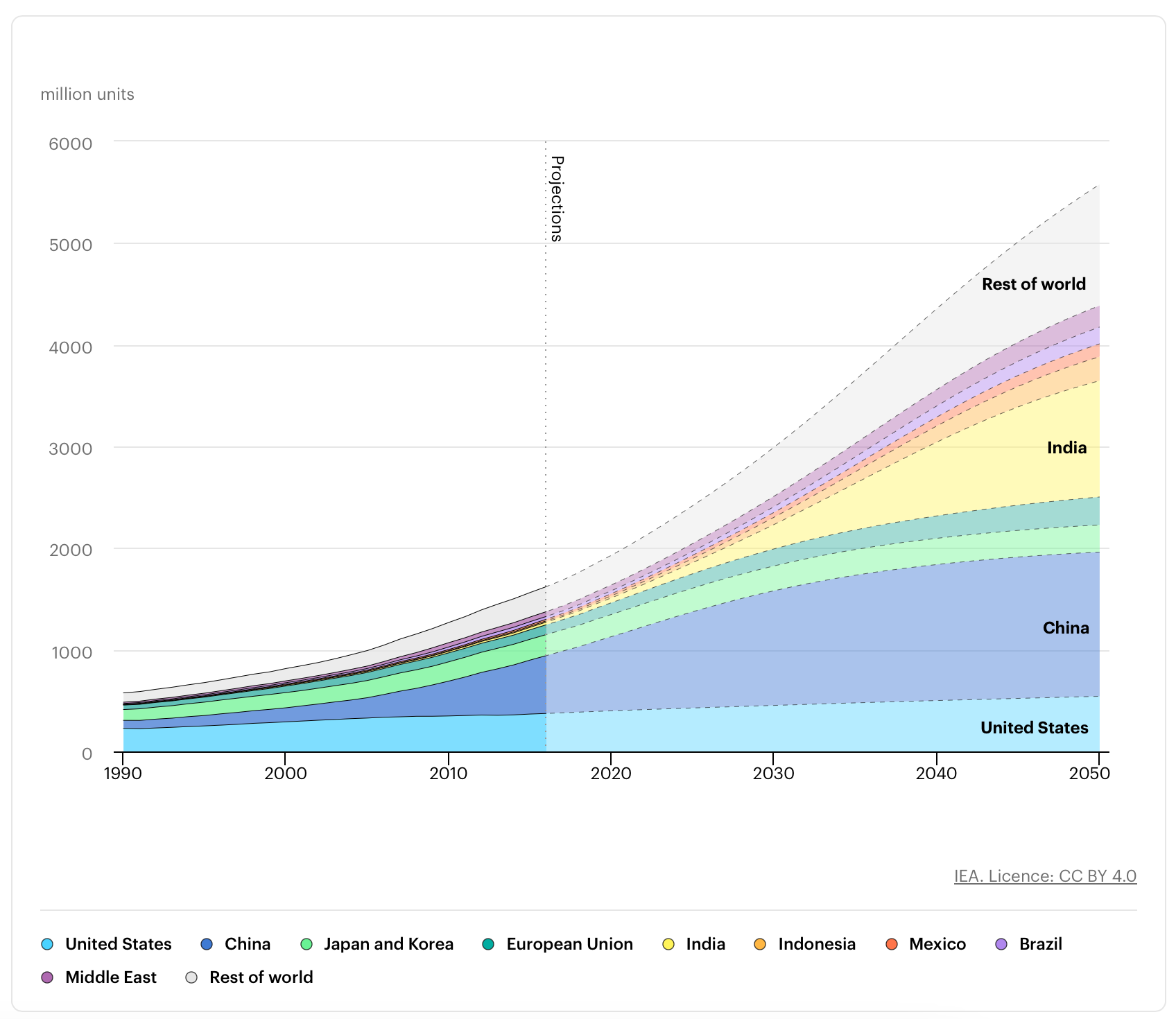
These data make a really clear case for us to put a wartime scale of resources into innovating and deploying low-cost, highly-efficient, and ideally circular heating and cooling systems around the world. But for those of us, like me, who live in a wealthier context with existing heating systems – many of which, at least in North American urban centres, depend on gas.
In case you hadn't noticed, the question of what becomes of that gas network has become something of a culture war.
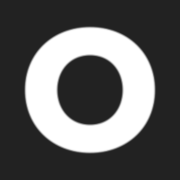
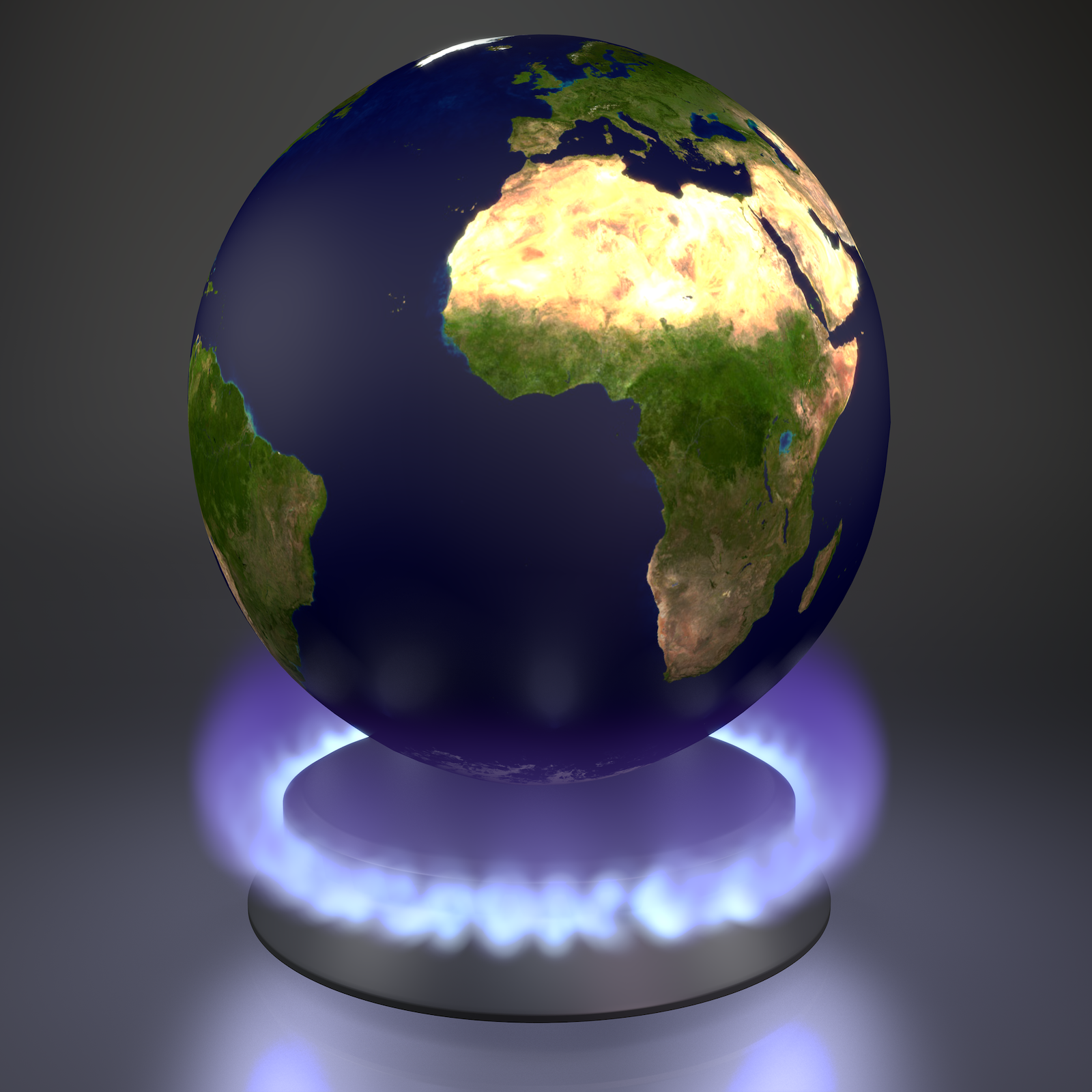
There are a series of very legitimate questions at the heart of what to do about an energy system which, as we all know, has negative climate change, as well as human health, and other, impacts.
Do we get rid of it all as quickly as possible?
Do we phase in less harmful forms, such as renewable natural gas (RNG), to replace some or all of our current gas usage?
Do we still use some of it as a transitionary or stop-gap fuel in certain while we wait for renewably-generated electricity to become more economical in all cases?
Do we force people to install, sometimes expensive, on-site renewable energy generation to buy-down the costs of upgrading the gird and large-scale generation?
Contemporary thermal energy networks, because of the way they're designed to cycle heat around to optimize efficiency (including, as I so love, using "waste" heat from businesses or other big producers) become attractive in answering this "what do we do" question for a few reasons.
Firstly, the latest generation designs of thermal energy networks are so efficient that they can do more (sometimes more than the current gas network) with less. By cycling heat throughout a series of diverse users, they effectively become an efficient thermodynamic commodity markets: you need heat? I need cooling. Let's trade!
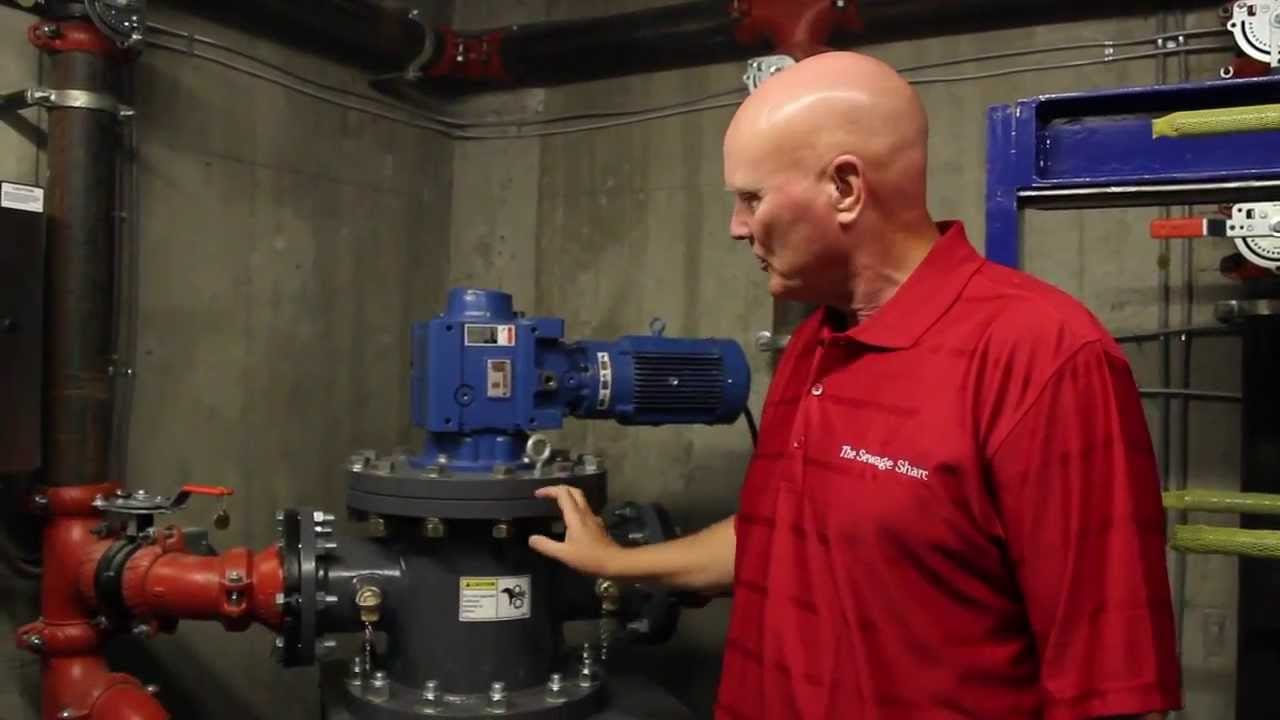
Secondly, because the backbone of a thermal energy network – pipes! – are familiar to most utilities, it's much easier for both the gas companies, and the people they employee, to turn their systems over into TENs. This recognition is the basis of the wonderful UpgradeNY Coalition's work to pilot, and eventually mandate, conversions of gas systems into TENs.
I had the privilege to meet and help host them in November of 2024, and in a series of dialogues we had with unions, utility leaders, and others, we all felt that:
- Thermal energy networks (TENs) represent a high-potential decarbonization solution, threading the needle on constraints related to affordability, justice for workers, and availability of electricity.
- Many current approaches to urban decarbonization (the "building by building" approach) are uniquely disadvantageous to existing, often unionized, utility and construction workers; TENs provide a common ground for unions to work with labour and environmental groups on a tangible environmental solution.
- Strategic partnerships across the value-chain in energy and construction, as well as with a range of environmental and community groups, can unlock economic and social benefits that go far beyond decarbonization.
Simply put, TENs aren't a silver bullet, but they look awful darn attractive for all of these reasons and more.
What I love about this is that we're already doing it. One company in Vancouver that I watch very closely, Creative Energy, is going to be launching three new networks in 2025/2026 alone. And they're not alone.
According to local thought-leaders Reshape Strategies, Metro Vancouver is a unique North American hub for this kind of system, with numerous public and private ones across the region.
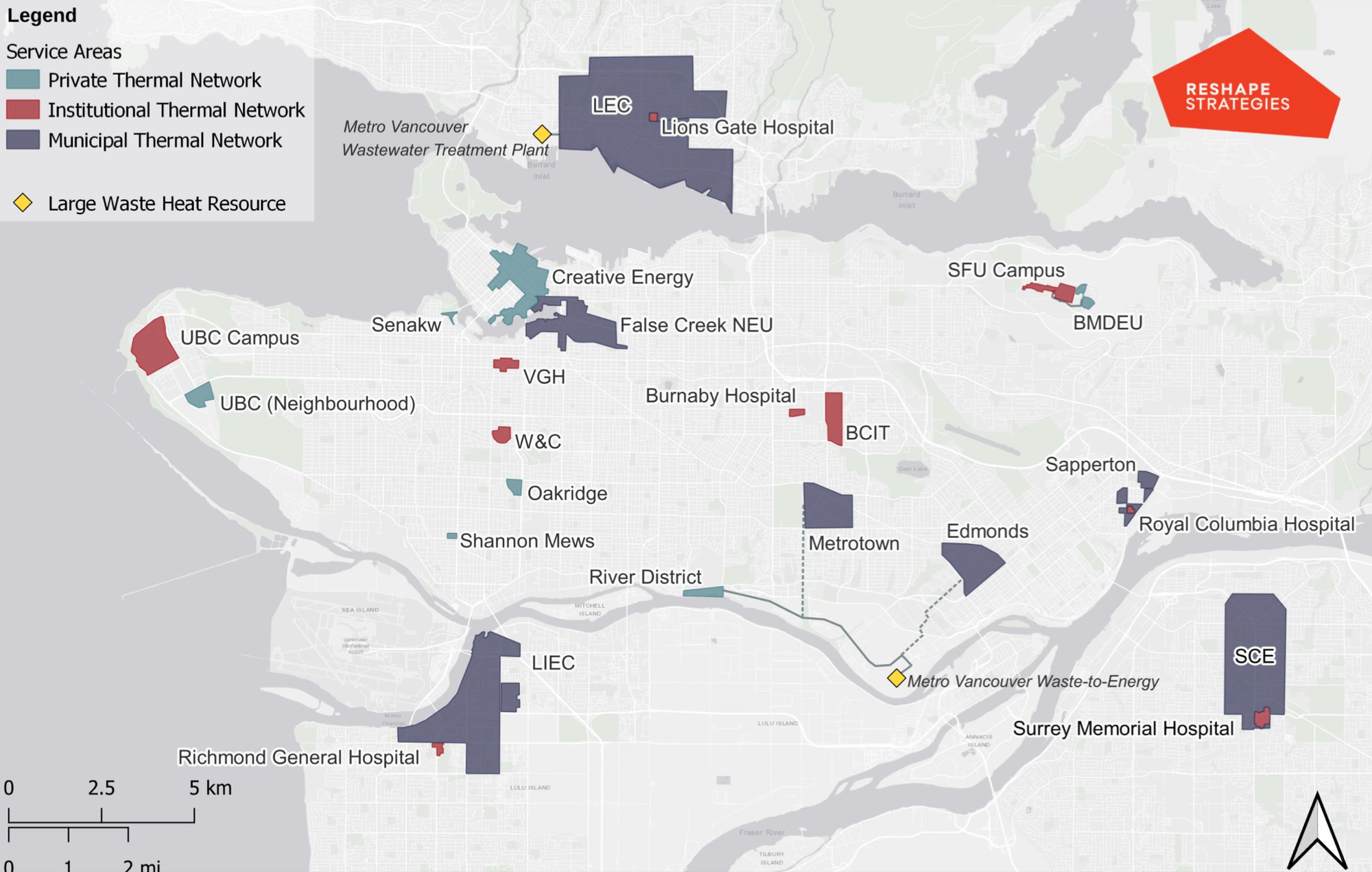
These systems, as they importantly note, already create more energy from all of the renewable natural gas (RNG) that's produced in BC, and will be significantly more by 2030.
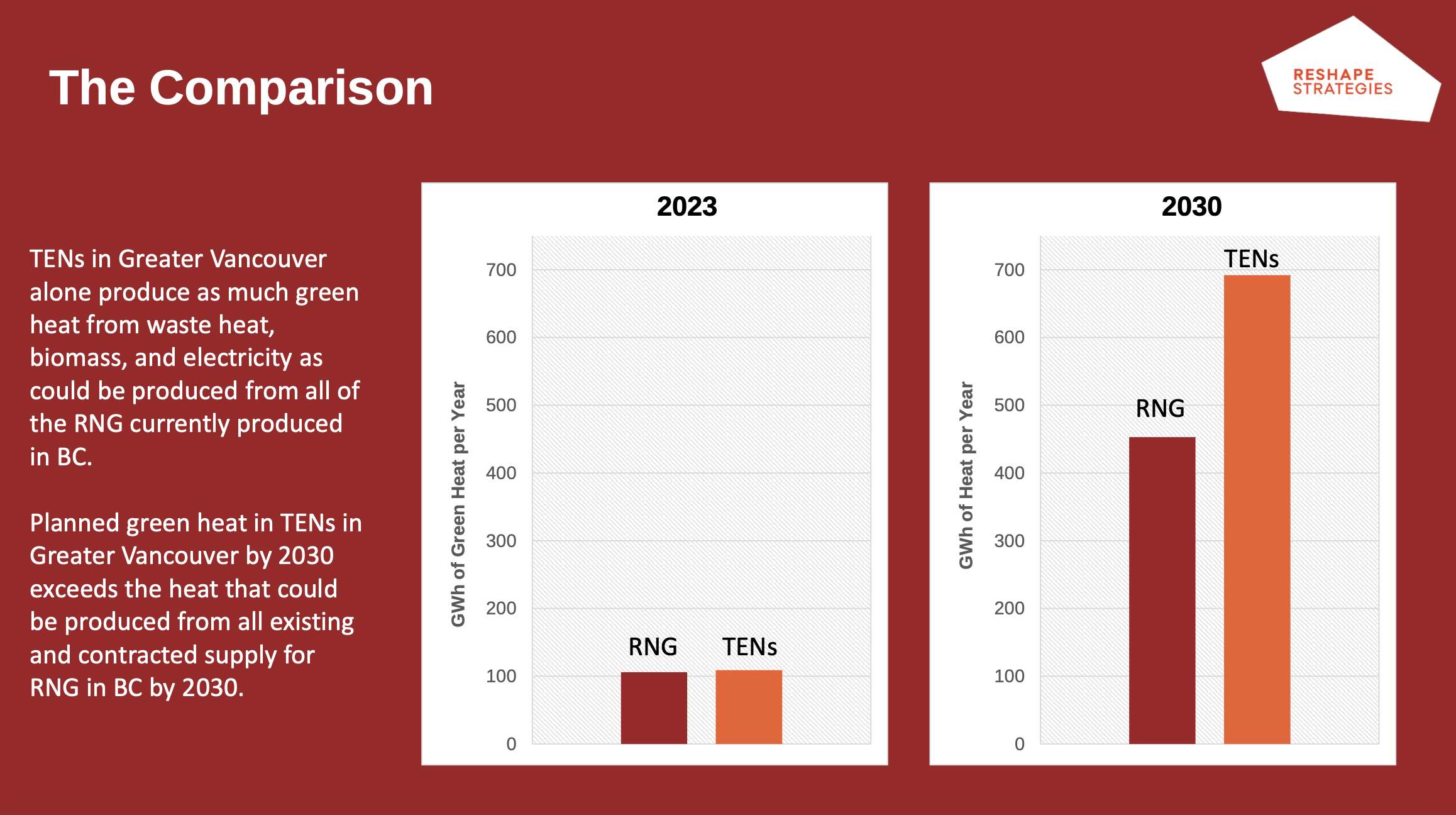
The stakes for this work couldn't be higher – the gas network is worth untold billions of dollars as it exists today – but the same is true for the opportunity. If gas companies have a meaningful off-ramp to participate in the renewable energy economy, not just meaningless handwaving about hydrogen boilers, then they can play a productive role. Right now, many view their only strategy as fight or die.
Perhaps the most exciting thing about something esoteric as thermal energy networks is that they're actually real, and you, as an everyday citizen, can see them and experience them. The City of Vancouver's sewer system gives numerous tours a year and, as the ARC facility in Copenhagen shows, these structures can be woven into the urban fabric in exciting and unique ways.
Stop by, check one out – the future isn't just around the corner, it might just be under your feet.
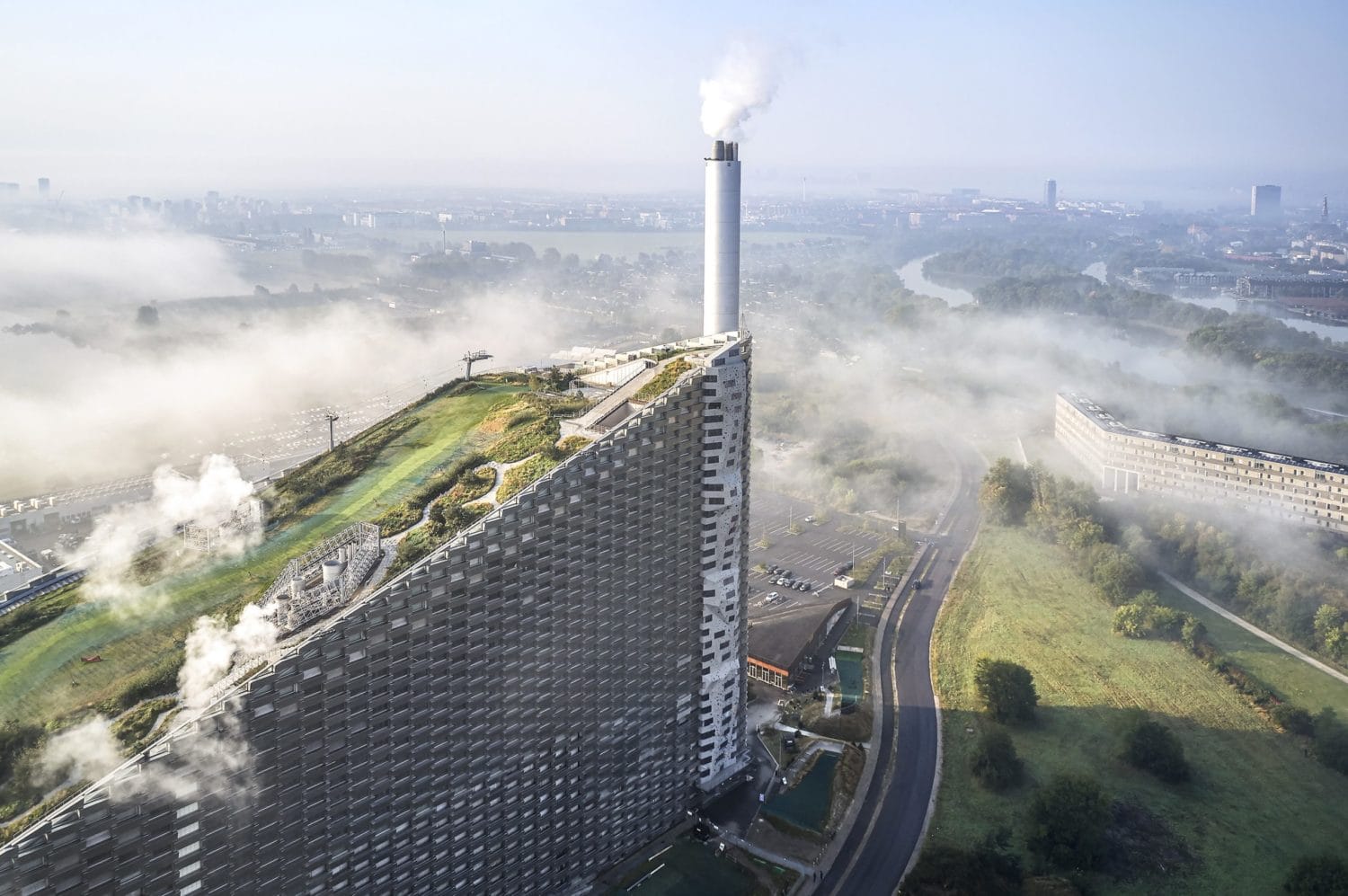
Sign up for George Patrick Richard Benson
Strategist, writer, and researcher.
No spam. Unsubscribe anytime.